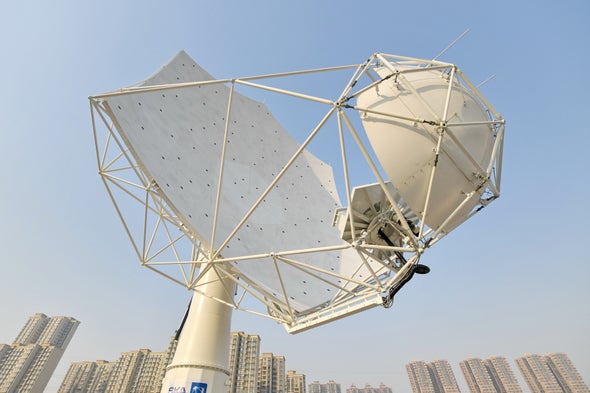
In the play Waiting for Godot, Samuel Beckett writes: “What are we doing here, that is the question. And we are blessed in this, that we happen to know the answer. Yes, in the immense confusion one thing alone is clear. We are waiting for Godot to come.”
What Beckett means by “Godot” is debatable. Throughout my career as an astronomer, I have waited for many “Godots,” including observational tests for my theoretical predictions. The most elusive of these involves observing the universe evolve in real time.
We often consider the cosmic microwave background, relic radiation from the early universe, as if it looked exactly the same three decades ago—when the COBE satellite first mapped it—to the way it looked recently—when observed by the Planck satellite. This is a valid simplification as long as the precision of our measurements is not good enough to notice the difference. But the reality is that the cosmos changes all the time, and so the properties of the cosmic photosphere—the original source of the microwave background originated, vary as well. Such cosmic change occurs very slowly over a timescale that is a hundred million times longer than a human lifetime. Can we notice the associated tiny variations over the decades that we monitor the sky?
The universe resembles a spherical archaeological dig centered on us. The deeper we look into space, the more ancient is the layer that we uncover. Owing to the finite speed of light, we see old images of distant sources that display the way they looked like a light-travel-time ago. If we allow more time to elapse, we see the same source at a later age. But in addition, when light travels towards us, its wavelength is stretched by the cosmic expansion. Visible light is shifted to the red, experiencing the so-called redshift. As Edwin Hubble realized about century ago, farther sources recede faster away from us, and so the redshift provides a measure of the recession speed of cosmological sources away from us.
We usually consider the redshifts of distant galaxies and other objects as a snapshot of the universe’s expansion at a particular time. But in fact, they are frames of a very slow-motion film, and we could in principle watch this film roll by waiting long enough. Can we actually detect a change in the cosmic recession speed of specific sources in real time? Allan Sandage reasoned in 1962 that it would be very challenging to measure such changes through observations of distant galaxies across the decades of an astronomer’s career.
In 1998, I wrote a paper suggesting a new approach for measuring the evolution in the expansion rate of objects at cosmological distances. The line of sight to distant quasars crosses numerous hydrogen clouds. Each of these clouds imprints an absorption feature on the observed spectrum of the quasar light. There are many more of these absorption features with measured redshifts than the number of galaxies along each line of sight. This offers a much better statistical sample for measuring the tiny redshift drift at each cosmic epoch.
By monitoring the slight drifts in the many redshifts marked by the rich forest of absorption features over a period of decades, one could potentially detect the evolution of the expansion rate of the universe in real time. The velocity shift is just below our best sensitivity in detecting planets by the tiny reflex motion of their host stars. A decline in the recession speeds of absorbing systems over decades of observations would imply cosmic deceleration whereas temporal growth would imply cosmic acceleration.
During the first half of the history of the universe, the cosmic expansion rate slowed down owing to the gravitational attraction imparted by radiation and matter. But during the second half, radiation and matter were diluted so much that the vacuum’s “dark energy” dominated and the cosmic expansion was accelerated by the vacuum’s repulsive force.
By monitoring the cosmic expansion in real time, we can observe the early deceleration at high redshifts and the late acceleration at low redshifts. The transition between the two phases would pin down the cosmic mass budget in terms of the relative proportions of dark matter—which dominates early on—and dark energy—which dominates later times. All in all, the redshift drift associated with the aforementioned Sandage-Loeb Effect can provide us with a direct view of how the universe expands as if we were watching it in a movie theatre. This view would become feasible with planned high-resolution spectrographs on the next generation of extremely large, ground-based telescopes.
The absorption featured imprinted by hydrogen atoms along the observing path to quasars stems from two facts: that hydrogen is the most abundant element in the universe, and that it can be excited from its ground state to the first energy level above it by absorbing an ultraviolet photon at a wavelength of 12.16 millionths of a centimeter, following the so-called Lyman-alpha transition. The absorption feature from a distant hydrogen cloud appears at a wavelength that was stretched by cosmic expansion since the time of absorption. Therefore, multiple clouds along the line of sight produce a forest of absorption features at different wavelengths, which looks like a weathered picket fence in the quasar spectrum, all the way to the Lyman-alpha wavelength at the source redshift. Transitions from the ground level of hydrogen to higher energy levels, labeled Lyman-beta, gamma, delta and so on, are less prominent.
Coincidentally, several of the above facts about hydrogen were discovered at Harvard University. The Lyman series is named after Theodore Lyman IV who directed the Jefferson Physical Laboratory a century ago and discovered experimentally these hydrogen transitions. Subsequently, Cecilia Payne-Gaposchkin discovered in the research for her Ph.D. thesis that the sun is made mostly of hydrogen; she served as chair of the Harvard Astronomy Department. Later, Edward Purcell, a Harvard physics professor and Nobel laureate, discovered the prominent hydrogen gas permeating the Milky Way galaxy by detecting its 21-centimeter transition. This notion was extended to the intergalactic medium by George Field, the first director of the Center for Astrophysics on the Harvard campus.
We are fortunate to inherit the path carved by these pioneering studies and to use the Lyman-alpha forest of hydrogen to inform us how the universe evolves in real time. Detecting redshift drifts, which could be possible within a decade or two with telescopes that are already under construction, such as the Square Kilometer Array and the Extremely Large Telescope, underline the most fundamental facet of our life: that everything around us changes, including the universe at large, and that we should endlessly adapt to new circumstances including cosmic loneliness as a result of accelerated expansion. The perpetual change on the cosmic scale is the slowest “Godot” that astronomers have a good reason to be waiting for.
This is an opinion and analysis article.
"time" - Google News
May 06, 2021 at 10:01PM
https://ift.tt/3nXIvRt
Watching the Universe Expand in Real Time - Scientific American
"time" - Google News
https://ift.tt/3f5iuuC
No comments:
Post a Comment